Metal Ductility and Temper: The Science Behind Softness and Strength
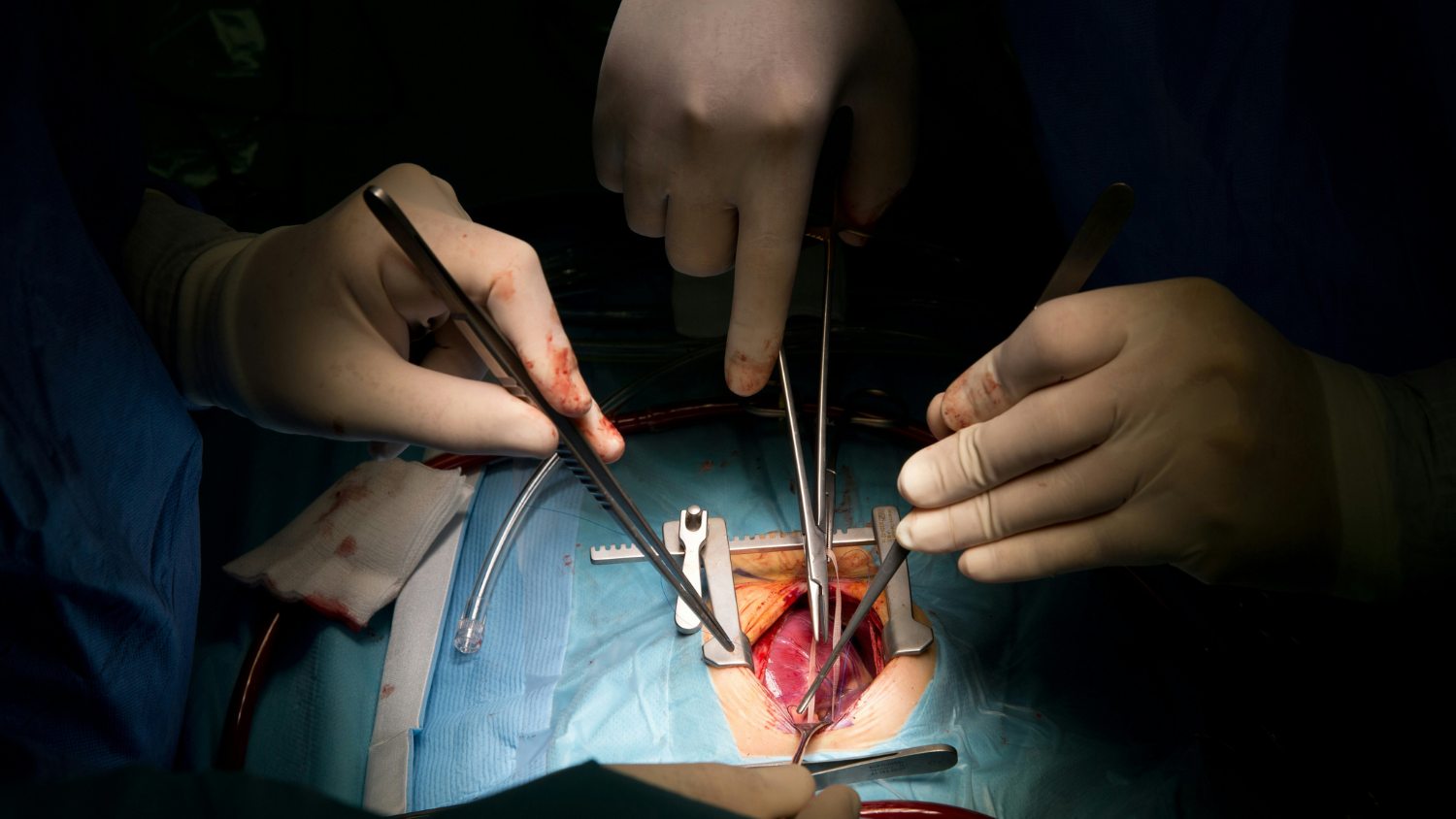.png?width=50)
Ductility is a key concept in materials science, describing a metal’s ability to deform under stress without breaking. Whether you’re designing cutting-edge aerospace components or testing the properties of innovative alloys, ductility plays a critical role in shaping how materials behave and perform.
But what gives metals their ductility, and how does the process of tempering - hardening or annealing - affect their atomic structure?
At Advent Research Materials, we supply metals for research and innovation, offering materials in a range of tempers to suit the needs of scientists and engineers worldwide.
What Is Ductility, and Why Does It Matter?
Ductility refers to how easily a metal can stretch or deform under tensile stress.
Metals like gold, copper, and aluminium, known for their high ductility, can be drawn into wires or shaped into complex forms without fracturing. This property is critical in industries such as construction, electronics, and medical technology, where precise shaping and reliable performance under stress are non-negotiable.
In research, ductility offers more than just practical utility. It provides insights into a metal’s internal structure, revealing how atomic arrangements respond to external forces.
Testing ductility, often through tensile tests, helps researchers optimise metals for real-world applications, from lightweight aircraft parts to robust medical implants.
How Tempering Affects Ductility
Metals don’t naturally exhibit the same level of ductility—they’re shaped by their processing history, known as temper. Temper refers to the heat treatment or mechanical work a metal undergoes, which alters its microstructure and, consequently, its properties.
The Science of Annealing
When a metal is annealed, it’s heated to a specific temperature and cooled slowly.
At the atomic level, this process allows the crystal lattice to rearrange, relieving internal stresses and creating a more stable structure.
- Grain Structure: Annealed metals develop larger, more uniform grains, which enhance their flexibility.
- Dislocations: Dislocations—atomic-scale irregularities—are reduced, making the material softer and easier to work with.
- Atomic Mobility: Atoms in annealed metals have more freedom to move, allowing for significant deformation without fracturing.
The result? Annealed metals are highly ductile and ideal for applications requiring extensive shaping, such as forming thin sheets or intricate wires.
The Rigidity of Temper-Hard Metals
In contrast, temper-hard (or work-hardened) metals are strengthened through mechanical deformation, like rolling or drawing, without significant heating. This process increases the metal’s strength but decreases its ductility.
- Grain Structure: Cold working elongates and distorts grains, creating a directional grain flow that enhances strength but makes the metal less flexible.
- Dislocations: The density of dislocations increases dramatically, “locking” the structure in place and resisting further movement.
- Atomic Mobility: Reduced atomic mobility makes these metals harder but more prone to cracking under stress.
Temper-hard metals are often chosen for structural applications where strength and resistance to deformation outweigh the need for flexibility.
Atomic Differences: Annealed vs. Temper-Hard
The atomic structure of a metal is the foundation of its properties. Annealing promotes a relaxed, uniform arrangement of atoms, resulting in a soft, pliable material. Temper-hard metals, by contrast, have a strained lattice with high dislocation density, creating a rigid, high-strength material.
These differences influence everything from a metal’s performance under load to its ability to withstand extreme conditions. For instance, annealed metals are commonly used in cryogenics due to their flexibility, while temper-hard metals excel in load-bearing structures.
Applications of Ductility and Temper in Innovation
Metals with carefully tailored ductility and temper play a significant role in driving advancements across a variety of industries:
Medical Devices: Titanium (Medical Grade) and its alloys are prized for their combination of ductility and biocompatibility. They’re essential in producing surgical implants, stents, and medical tools that need to withstand shaping without compromising strength. For example, titanium is widely used in orthopaedic implants.
Energy Sector: Copper’s ductility and excellent conductivity make it indispensable for renewable energy applications, from wind turbine wiring to solar power infrastructure. The ability to form copper into thin, highly conductive wires ensures reliable energy transmission in renewable systems.
Aerospace: Lightweight alloys with optimised ductility and strength are critical for aerospace components. Aluminium-lithium alloys, for instance, are used in aircraft fuselages and wings, where forming capabilities and resilience are equally important.
Supporting Researchers with Precision Metals
Ductility and temper are the keys to unlocking the potential of metals in research and innovation. By understanding how atomic structures respond to stress and treatment, researchers can design materials that perform better, last longer, and push the boundaries of what’s possible.
At Advent Research Materials, we understand the importance of having metals with precise properties, whether you need maximum ductility or optimal strength.
We supply a wide range of materials in both annealed and temper-hard conditions, enabling researchers to explore the full spectrum of metal performance.
Our metals come in various forms—sheets, rods, foils, and powders—tailored to meet the specific needs of experimental setups. Whether you’re studying atomic-level behaviours or developing new technologies, our materials can help you achieve your goals.
Ready to explore the metals we offer? Visit our catalogue or contact us to discuss your project.