Research Materials for Fusion Energy Research
The race to harness fusion energy - the process that powers the sun - has driven global research into high-performance materials capable of withstanding extreme heat, radiation, and plasma interactions.
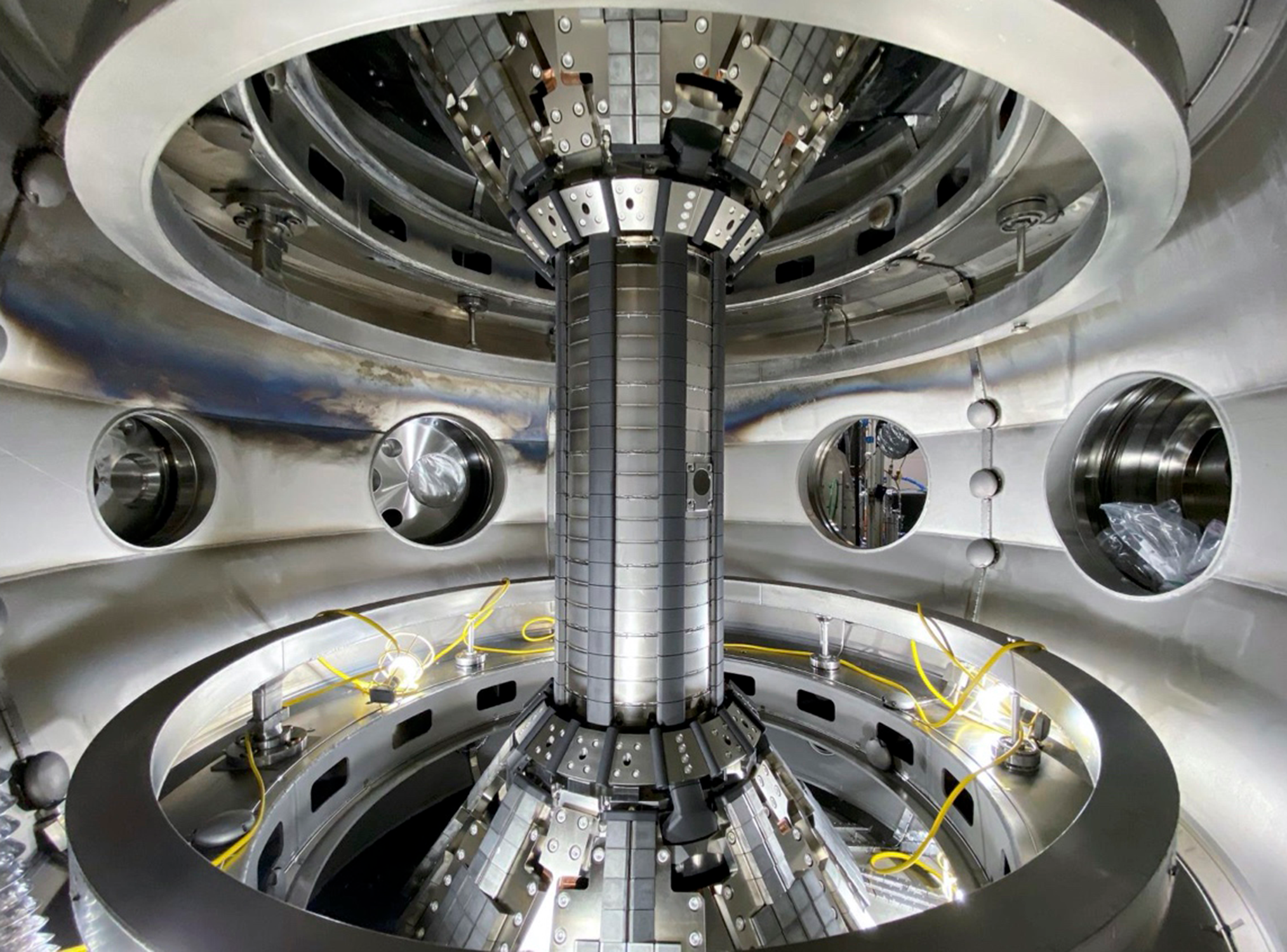
Research Materials for Fusion Energy Research
Unlike conventional nuclear fission, which relies on splitting heavy atomic nuclei, fusion generates energy by fusing light atomic nuclei, offering a virtually limitless and clean energy source.
However, the extreme conditions inside a fusion reactor present unprecedented materials challenges, requiring metals and advanced materials engineered for durability, stability, and efficiency.
At Advent Research Materials, we supply high-purity metals, alloys, and polymers essential for fusion research applications.
Our materials support critical areas such as plasma-facing components, hydrogen isotope containment, and structural reactor materials, enabling scientists and engineers to push the boundaries of fusion technology.
Key Challenges in Fusion Energy Materials
Fusion reactors, including experimental facilities such as ITER (International Thermonuclear Experimental Reactor), require materials that can endure the following conditions:
- Extreme Temperatures – Plasma in a tokamak or stellarator can exceed 100 million degrees Celsius, demanding materials with high melting points and thermal stability.
In fact, some experimental fusion reactors, such as JET (Joint European Torus) and ITER (International Thermonuclear Experimental Reactor), aim to sustain plasma at temperatures above 150 million degrees Celsius - which is even hotter than the core of the sun! These extreme temperatures are necessary because fusion reactions require hydrogen isotopes (deuterium and tritium) to overcome their natural electrostatic repulsion. The hotter the plasma, the more likely these particles are to collide and fuse, releasing energy.
- High Radiation Exposure – Neutrons produced in fusion reactions cause material degradation, necessitating radiation-resistant metals and composites.
- Hydrogen and Tritium Management – Materials must effectively contain and manage hydrogen isotopes (deuterium and tritium), key fuels for fusion reactions.
- Plasma-Facing Components (PFCs) – The reactor’s inner walls must resist erosion, embrittlement, and plasma interactions, while maintaining thermal conductivity.
Tokamaks and Fusion Research in Oxford
What is a Tokamak?
A tokamak is one of the most advanced designs for achieving controlled nuclear fusion.
It is a doughnut-shaped (toroidal) chamber that uses powerful magnetic fields to confine and stabilise superheated plasma, allowing fusion reactions to occur. The goal is to replicate the same process that powers the sun, where hydrogen isotopes (deuterium and tritium) fuse at extreme temperatures, releasing enormous amounts of energy.
Inside a tokamak, hydrogen isotopes (deuterium and tritium) are heated to extreme temperatures, forming a plasma where fusion occurs, releasing vast amounts of energy.
The key challenge is keeping the plasma confined and stable for long enough to sustain energy production. Magnetic fields, generated by external coils, form a barrier that prevents the plasma from touching the reactor walls, maintaining the extreme conditions necessary for fusion. This energy can then be converted into electricity, offering a cleaner and more sustainable power source compared to conventional nuclear fission.
A variation of this design, the spherical tokamak, has a more compact, cored-apple shape rather than the conventional ring doughnut. This design is being explored for its potential to improve efficiency, enhance plasma stability, and reduce the size of future fusion reactors.
For a deeper insight, watch this Tokamak Energy video explaining tokamak designs and the advantages of spherical tokamaks.
Fusion Research in Oxford: Advancing the Future of Energy
Oxford has become a global hub for fusion energy research, with cutting-edge developments led by companies such as Tokamak Energy and First Light Fusion.
These organisations are pioneering advancements in compact fusion reactors and alternative fusion approaches, driving innovation in plasma confinement, neutron shielding, and high-performance materials.
With major research initiatives and private-sector investment accelerating progress, Oxford is shaping the future of commercial fusion power and demonstrating the potential for clean, sustainable energy on a large scale, with cutting-edge developments led by companies such as Tokamak Energy and First Light Fusion.
Metals and Alloys for Fusion Energy Research
To meet these challenges, high-performance metals and alloys play a crucial role in fusion reactor components:
- Tungsten (W) and Tungsten Alloys
Used for: Plasma-facing components (PFCs), divertors, and first-wall materials.
Why? Tungsten has an exceptionally high melting point (3422°C), low sputtering yield, and excellent thermal conductivity, making it ideal for handling extreme heat and neutron bombardment.
- Copper (Cu) and Copper Alloys
Used for: Heat exchangers, cooling systems, and superconducting coils and magnets.
Why? Copper provides high thermal conductivity and efficient heat dissipation, critical for reactor cooling systems and high-power magnet technology. These magnets are essential for confining plasma within fusion reactors. A notable example is the use of rare-earth barium copper oxide (ReBCO) materials, such as yttrium barium copper oxide (YBCO), which exhibit superconductivity at relatively higher temperatures. This property allows for the creation of stronger magnetic fields necessary for efficient plasma confinement.
- Nickel (Ni) and Nickel Alloys
Used for: Structural components and hydrogen isotope management.
Why? Nickel alloys exhibit high-temperature strength and corrosion resistance, essential for long-term reactor performance.
- Zirconium (Zr) and Zirconium Alloys
Used for: Tritium breeding materials and reactor structural components.
Why? Zirconium has low neutron absorption cross-section, making it suitable for fusion neutron environments.
- Tantalum (Ta) and Niobium (Nb)
Used for: High-performance structural materials in extreme environments.
Why? These refractory metals offer superior resistance to heat, corrosion, and neutron damage.
Hydrogen and Tritium Management: Materials for Isotope Containment
Fusion reactions rely on deuterium and tritium isotopes as fuel, making hydrogen-permeable and containment materials crucial for efficiency and safety. High-performance metals for tritium processing include:
- Palladium (Pd) and Palladium Alloys – Used in hydrogen purification membranes due to high selectivity for hydrogen isotopes.
- Vanadium (V) and Vanadium Alloys – Demonstrate high hydrogen permeability and low neutron activation, making them suitable for tritium extraction systems.
- Iron-Chromium-Aluminum (Fe-Cr-Al) Alloys – Offer high-temperature oxidation resistance, ensuring long-term durability in tritium environments.
Advanced Ceramics and Composite Materials for Fusion Applications
Beyond metals, ceramics and composites play a vital role in insulating, shielding, and structural applications within fusion reactors:
- Beryllium (Be) – A lightweight metal used as a neutron multiplier in tritium breeding systems. While not a ceramic, beryllium oxide (BeO) is sometimes utilised in high-temperature applications for its thermal and electrical insulating properties.
- Alumina (Al₂O₃) and Zirconia (ZrO₂) – Employed in thermal insulation and electrical shielding.
- Silicon Carbide (SiC) Composites – Being explored for radiation-resistant structural components due to low neutron activation and high-temperature strength.
Why Choose Advent Research Materials for Fusion Research?
- High-Purity Materials – We supply research-grade metals, alloys, and ceramics engineered to withstand extreme fusion conditions.
- Diverse Product Range – From plasma-facing materials to isotope management solutions, we offer a comprehensive selection of fusion-compatible materials.
- Trusted by Leading Researchers – We supply academic institutions, energy research facilities, and industry pioneers in plasma physics, nuclear materials, and high-energy applications.
Enabling the Future of Clean Energy
Fusion energy has the potential to redefine global power generation, offering a virtually limitless supply of clean, sustainable energy. By supplying critical materials for fusion reactor development, Advent Research Materials is proud to support scientists, engineers, and energy pioneers working towards commercial fusion power.
Explore our range of high-purity materials and contact our team to discuss your specific requirements.
Product Search
Advent has over 1000 product lines and over 10,000 catalogue items, consisting of 55 different metals and alloys. Our range of online materials includes: Pure metals, Alloys and Polymers. Metal purities typically range from 99.0 to 99.999%.
Search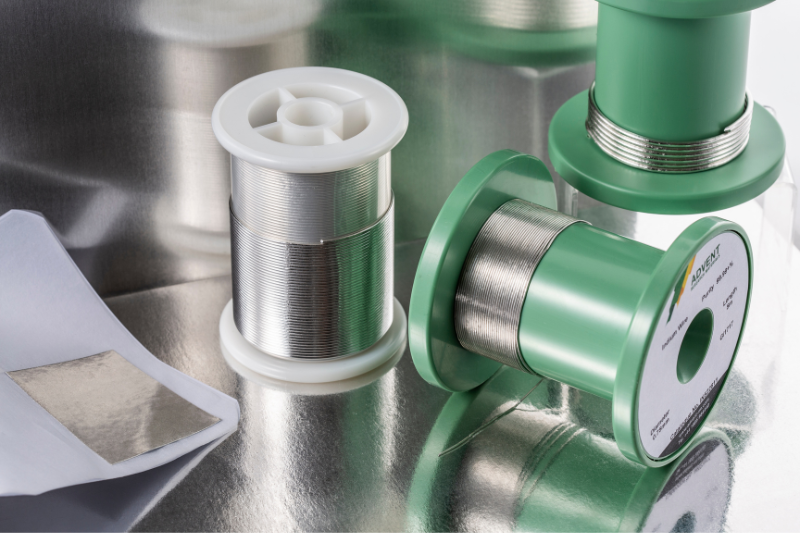.png?width=50)
Material Recycling
To help reduce waste and promote sustainability, we are introducing Advent ReMaterial—a dedicated, free-of-charge recycling initiative for scientists, researchers, and all our customers.
More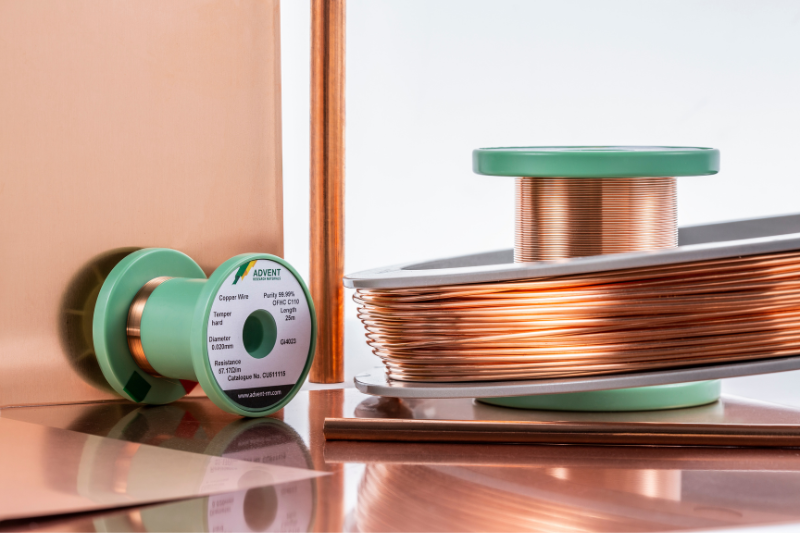